Surprising Twist to Protein Misfolding Discovered
Post# of 63824
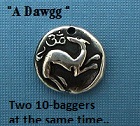
Surprising Twist to Protein Misfolding Discovered
Jan. 14, 2013 — An effort to develop software that unravels the complexities of how proteins fold is paying dividends in new findings on how they misfold, according to researchers at Rice University.
A three-dimensional plot of energies in multidomain protein folding, produced at Rice University with the AWSEM-MD program, shows stable regions in blue at the bottom (“N”) for the protein in its correct native state, and at the top (“I”) in its incorrect, misfolded state. Both extremes are equally stable, though “N” proteins are lower in energy. Red and green represent the separate protein domains; the colors are jumbled in the domain-swapped structure (top) and in the "self-recognizing" structure (right), which have misfolded due to strong self-recognition interactions among the short residue sequences shown in the colored stripes. Researchers suspect misfolded proteins play a major role in the aggregation of amyloid fibers implicated in degenerative diseases
The study published this week in the Proceedings of the National Academy of Sciences by chemist Peter Wolynes and his team at Rice's BioScience Research Collaborative should be of particular interest to those who probe the roots of degenerative diseases associated with the aggregation of amyloid fibers in the body. These include Alzheimer's and Parkinson's diseases and Type 2 diabetes.
The molecular dynamics software to predict how strands of residues bend and twist into their functional shapes is designed to follow Wolynes' and his colleagues' groundbreaking "principle of minimal frustration." These residues, the molecular beads that make up proteins, follow the path of least resistance as they fold into their native states. The principle describes how evolution has shaped the path a protein takes toward stability.
The software, called AWSEM-MD (for associative memory, water-mediated structure and energy model) simulates the possible ways beads in a strand should fold, based on the energies at play down to the submolecular level, and accurately predicts the final structure. Two developers of the current version, Weihua Zheng, a postdoctoral researcher at Rice, and Nicholas Schafer, a graduate student, are co-authors of the new paper, the latest in a series on folding dynamics dependent on the software.
The researchers set out to confirm that a process seen by experimentalists called domain swapping is one cause of protein misfolding. Domains are conserved parts of protein chains. Occasionally, a domain in one chain may encounter its doppelgänger in a nearby chain and become entangled with it via interactions similar to those in the correctly folded state.
The result is often a dimer -- a kind of protein Siamese twin -- that probably won't be able to perform its intended biological task and may become part of a damaging amyloid fibril. "Experimentalists had some strong laboratory evidence that dimerization is a consequence of minimal frustration, an idea proposed earlier by our group on more general grounds," Wolynes said. "So we figured it would be nice to do a simulation to check it."
The team did indeed see domain swapping in their models of human cardiac titin, a muscle protein. But they were surprised to see something they weren't looking for: evidence that identical sequences in neighboring chains, as short as five to seven residues, had the unfortunate tendency to find each other and stick together.
They found instances of such "self-recognition" tipped the balance of energies that dictated whether a protein would fold properly. Replacing just a few residues in one fragment eliminated self-recognition and lowered the incidence of domain swapping, Wolynes said.
"We weren't the people who thought of this as a possibility," he said. "It had been suggested by others, although I never really believed it because it doesn't have an obvious connection to the principle of minimal frustration." But the simulations showed instances where sticky self-recognition in one segment of a chain could affect the energy of residues down the line and effectively introduce "frustration" that keeps the rest of the protein from folding at all and results in high disorder, or entropy.
While the models don't directly connect to the formation of amyloid fibrils, Wolynes said, anecdotal evidence indicates protein-folding diseases have some correlation with fevers that allow the extra entropy to stabilize the misfolded forms. "Our results would provide a new explanation," he said, for how a disordered part of the chain can contribute to the stability of these misfolded states at high temperature.
"When you hear 'take two aspirins and call me in the morning,' your doctor is doing you a bigger favor than you know," Wolynes said.
The discovery could open paths for researchers to design drugs that inhibit specific interactions. "Very minor changes seem to destroy this self-recognition in the computer simulation, and that's what we want the experimentalists to do: Make those changes to see if they decrease the self-recognition effect," he said.
"Our simulations provide structural details of misfolded proteins at the molecular level that are difficult for experiments to probe," Zheng said. "These can generate specific hypotheses they can test."
The researchers hope their work will be useful to both experimentalists and other computational protein-folding researchers.
"AWSEM is hosted on Google Code, which requires all code to be open-source," Schafer said. "So it's available to anyone who wants to use it. What we're seeing with these studies is that the values we get by applying the principle of minimal frustration are appropriate globally, not just for predicting the native structures of proteins. It can predict bound structures (like dimers) and misfolded structures as well.
"You always have to be careful about using models that 'rig the deck' in favor of a particular anticipated result," he said. "But what's interesting is that our model doesn't have any information a priori about these specific types of misfolded structures. Our model is parameterized using as input only experimental data for properly folded structures and then applying the principle of minimal frustration. The wide range of successes we've had this year tells me that we have a decent method for deriving the strengths of the interactions."
"We never really thought about specific kinds of misfolding or the aggregation process when we built our model around the principle of minimal frustration," Zheng said. "But they all fall into place."

