(Total Views: 571)
Posted On: 01/25/2017 5:05:15 PM
Post# of 72447
The blog shows the cover of the Newsweek magazine. Here's the p53 article in it:
THE CANCER KILLER
In other families, as generation gives rise to generation, father passes to child a cleft in the chin, a love of music; mother passes to child an aptitude for dance, a curve of the cheeks. In one family, the legacy was far different. What was being handed down, unknowingly, was cancer. Or, more precisely, the mutant form of a gene whose inheritance virtually guarantees that a malignant tumor will grow. Grandfather carried the mutation. He developed pancreatic cancer at the age of 48; he died two years later. But first he passed on the mutant gene to his five children. One daughter developed breast cancer at 22 and thyroid cancer at 34. A son had a brain tumor at 54. Another daughter was diagnosed with breast cancer at 32; a year later she was dead. And that was only the first generation.
For as long as biologists have studied cancer, they have nurtured one dream. Their hope has been that, for all of the many different cancers that can eat away at a body--from the glioblastomas that creep through the brain like kudzu through Georgia, to the lung and colon cancers that envelop vital organs like a death shroud--there will turn out to be a single step that determines whether cells turn cancerous. By targeting drugs at that step, physicians would be able to stop a cell from heading down the path toward cancer just as a switchman stops a train from going down the wrong track. It turns out that a gene called p53--the gene whose useless, mutant form the luckless family passed from parent to child through the generations--may be that switch. If p53 is healthy and doing its job, it keeps the cell on the track of normalcy. But if the p53 gene is absent, damaged or tied up by other molecules, the cell goes down the track toward cancer. "p53 fulfills this long-held hope," says Dr. Bert Vogelstein of the Howard Hughes Medical Institute (HHMI) and the Johns Hopkins Oncology Center, who found, in 1989, that some 80 percent of colon cancers involve p53. "Alterations of [the p53 gene] appear to be involved, directly or indirectly, in the majority" of human cancers. All told, p53 has been implicated in more kinds of cancer than most people knew existed: 52 and counting.
Not bad for a gene that first broke on the scene in a bad case of mistaken identity. In 1979, David Lane of the University of Dundee in Scotland and Arnold Levine of Princeton University independently discovered p53, the protein that the p53 gene makes. In 1982 biologists isolated the p53 gene. But it seemed to cause, rather than suppress, cancer. Few researchers were interested in yet another one of those. It was not until 1989 that Levine and Vogelstein separately discovered p53's true colors: it was a tumor killer. With this realization, some 2,000 biologists have now dropped the date they brought to the dance--their previous research subject--and latched onto the pretty new arrival. "Our interest converged on p53 like no other molecule," recalls oncologist Richard Elledge of the University of Texas Health Science Center at San Antonio. Three years ago p53 won the science world's Academy Award, earning "Molecule of the Year" honors from the journal Science. Just last month researchers led by Nikola Pavletich of New York's Memorial Sloan-Kettering Cancer Center produced a stunning photo of p53 (above) that shows how rogue molecules can prevent it from performing its good deeds. Today there are more than 5,200 published studies on p53, and biologists are convinced that p53 is bound for even greater glory. "What I like so much about p53 is that it predicts ways to treat cancer," says Princeton's Levine. "For the first time, we can honestly translate from the lab to the clinic."
p53 acts as the cell's director of damage control. A healthy cell, usually, keeps a small number of p53 proteins around, continuously degrading them and replenishing the supply. But if something--ionizing radiation, a chemical carcinogen, chemotherapy drugs--damages a cell's DNA in a way that threatens to set it on the path to cancer, the cell switches into high alert. If everything is working right, something signals the p53 to stop degrading "and tells it that it's time to be active," says molecular biologist Carol Prives of Columbia University. The p53 supply builds up. p53 starts acting like an office clerk who, discovering a typo in an original document that is about to be copied, turns off the copier until he can fix the typo. p53 turns off the cell's copying machinery until the cell can repair its damaged DNA. To do this, p53 floats toward the cell's genes (diagram, page 46). It slips into a specific stretch of DNA. There, it triggers expression of genes nearby. These genes make proteins that directly inhibit growth of the cell. The tumor-to-be is stopped dead. Sometimes, though, p53 acts more like the clerk so disgusted with the many typos that he just trashes the document: p53 activates the cell's suicide software.
Except when it doesn't. Even good genes can go bad, and the gene that makes p53 has several ways of failing. Those failures account, directly or indirectly, for an estimated 60 percent of human cancers, including those of the breast, lung, liver, skin, prostate, bladder, cervix and colon (chart, page 45). Most often, the p53 gene goes bad by undergoing a mutation, typically a spelling mistake (FAQs, page 50). One of the 2,362 chemical "letters" (designated A, T, G and C) that make up the p53 gene changes into another letter. When the p53 gene contains such a spelling mistake, the p53 protein that the gene makes is garbled, too. And proteins are not very forgiving of errors. A single wrong letter in a crucial part of the p53 gene produces a protein with a wrong molecule; the protein is now no more able to suppress tumors than a cork is to cap a volcano.
The daughter with both breast and thyroid cancer married. It would be years before Drs. Frederick Li, of the Dana Farber Cancer Institute in Boston, and Joseph Fraumeni, now at the National Institutes of Health, would discover the silent killer--a mutated form of the p53 gene--in each and every one of her cells. Including her egg cells. She bore two daughters and a son. She passed the mutant p53 gene to both daughters. Her little boy, through the dumb luck that biology sometimes tosses up, was spared. The first little girl developed a soft-tissue cancer in her abdomen at the age of 1; she didn't live to see two candles on her birthday cake. The second daughter developed a soft-tissue cancer in her thigh, at 3.
The Li-Fraumeni families (the syndrome is named after its discoverers) inherit their p53 mutations. If the sperm or egg from which a baby grew held a mutant p53, then every single cell of the 30 trillion in her body will also harbor a mutant copy. In theory, inheriting only one mutant p53 gene, from one parent, should not be a problem as long as the child inherits a healthy p53 gene from the other parent. The healthy copy should make enough p53 to keep tumors at bay. But p53 doesn't work that way. First of all, each cell with one bad p53 gene is only "one mutation away from completely lacking the function of this critical gene," explains p53 researcher Tyler Jacks of HHMI and the Massachusetts Institute of Technology. That mutation can occur when the cell--in the liver, lungs or any other organ--makes a spelling mistake as it copies its genes before dividing into two cells. Then the cell has lost its primary defense against cancer.
But even a cell whose healthy p53 gene stays that way can be in trouble. The p53 proteins made by the genes, both good and bad, get together in groups of four to form a sinuous complex that looks like ribbon on a Christmas present. If the mutant gene is churning out mutant proteins, then each four-ribbon tangle likely has a mutant among its strands. That is enough to keep the p53 ribbon from binding to DNA and halting tumor growth.
Most p53 mutations, though, are not inherited. Instead, they arise from a copying error or an attack by a carcinogen. Bulky chemicals, such as the benzopyrene in cigarette smoke, change G to T and C to A, for instance. A single mutant gene is enough to leave a cell with no healthy, tumor-quashing p53. And just one out-of-control cell can give rise to a deadly tumor.
The only son seemed to have evaded the family legacy. But his daughter developed leukemia by the age of 5; she died soon after her 6th birthday.
THE RUSH TO STUDY P53 has left pioneers in the field with divided feelings. On the one hand, says Princeton's Levine, "it is very hard to do original experiments when there are so many smart people going after the same thing. It can be dispiriting. In 1989, when Vogelstein found so many colon cancers had p53 mutations, I moved my whole lab into p53 research. But two years ago I started half of them on different projects; it's easier to be novel in new areas. And besides, p53 is in very good hands now." And in an unusual situation for a hot field with "Nobel" written all over it, those hands are cooperating. "People share [materials] even before they publish, which is very unusual," says Moshe Oren of Israel's Weizmann Institute. "But because it's so hard to keep up with the scientific literature, you have the embarrassing situation of rediscovering something that was discovered two years before."
Still, better rediscovery than none at all. Recently researchers have focused on how p53 cancers tend to be especially aggressive. They are more often fatal than cancers in which p53 is healthy. Part of the reason may be that a mutation can do worse than simply cripple p53. Levine and colleagues find that p53 can undergo "gain of function" mutations: they actually stimulate cell division rather than suppress it. The mutant seems to turn on a new set of genes, such as those that make a cell immune to cancer drugs.
A truism of oncology holds that cancer leaves no calling card. It is thought to be impossible to know for sure what started a tumor--secondhand smoke inhaled in a lobby, a virus contracted from one's first lover. p53 promises to change that. By examining the p53 genes in a tumor biopsy, researchers can, in some cases, trace the disease back to its cause. As UT's Elledge puts it, "p53 can... be used as an archeological tool to tell us about past molecular skirmishes that touched off a cancerous war." The body count:
Cancer-causing benzopyrene in cigarette smoke primarily changes just one letter, at one spot, on the p53 gene: a G becomes T. Other compounds in smoke change C to A. In October, Texas researchers detected benzopyrene clinging right to the mutational "hot spot" that is mutated in lung cancer. "This is the closest to a smoking gun linking smoking to cancer that people have gotten," says molecular biologist Stephen Friend of the Fred Hutchinson Cancer Center in Seattle. The mutation robs the lung cell of its primary tumor-suppressing machinery. But cancer is not inevitable--"only" about 14 percent of cigarette smokers develop lung cancer. The lung cell must take one more hit before it starts growing wildly.
Two leading causes are the hepatitis-B virus and aflatoxin, a mold that grows on wet corn and other crops. Both work through p53, but in different ways. Aflatoxin causes a mutation by changing one G to a T; if the liver cell starts proliferating out of control, a hepatocarcinoma develops. The virus acts differently. It makes a protein that lurks near where p53 is made. Then it imprisons p53 and keeps it from reaching the double helix in time to stop proliferation.
If a skin cell's DNA is damaged by sunlight, p53 makes it commit suicide. But if ultraviolet light also causes a spelling mistake in the p53 gene (CC to TT), the damaged cell can proliferate unchecked.
When the human papillomavirus (HPV) sneaks into a cell of the cervix, usually during intercourse, it churns out virus proteins. One protein literally digests p53, turning it into a useless mush.
Researchers were baffled to discover that, in many breast cancers, the p53 gene looked perfectly healthy. But clearly it was not doing its job of stopping, or killing, proliferating cells. It turns out that, in at least some cases, a miscreant protein traps p53, explains Princeton's Levine. P53 can't get anywhere near the DNA that it needs to reach to save the cell. (Interestingly, during lactation of breast cells p53 is sent into exile to the cytoplasm; that way, they can proliferate enough to nurse a baby.) It is not known how often the trapping of p53 allows malignant breast cells to grow, but in another kind of cancer it is fairly common. In some 30 percent of soft-tissue tumors called sarcomas, a protein called MDM-2 snares p53 like a tuna in a net. "MDM-2 forms tight complexes with p53," says Moshe Oren, "and inactivates it."
Stephen Friend suspects that the fingerprints of other chemicals, from pesticides to industrial pollutants, will eventually be found on p53, answering how often pollutants cause cancer. Still, given the choice between knowing where a cancer came from and stopping it from going where it's going, everyone would choose the latter. Over the last two years, researchers have been making headway in using p53 to diagnose cancer early enough to allow lifesaving surgery, to determine the best therapy and--since no saga of cancer research would be complete without this-- to cure it.
The first sign of bladder cancer is often blood in the urine. That alerts doctors to screen the urine for abnormal cells. But they often do not find any until the cancer is too far along to treat. Researchers led by Hopkins's David Sidransky managed to use p53 to diagnose Hubert Humphrey's bladder cancer in a urine sample that had been stored since 1967 (Humphrey died in 1976). Using a genetic probe, they found, in a 1994 experiment, that cells in the urine contained a p53 mutation. If the diagnosis had been made in 1967, says Sidransky, it "could have changed the course of political history." Sidransky estimates that a quick and accurate urine test for bladder cancer, based on p53, is only two or three years away. Detected that early and treated--sometimes simply by nipping out the malignant cells--the cure rate is at least 90 percent.
The state of the p53 gene in a tumor can dictate the best course of treatment. For years oncologists assumed that radiation kills cells directly, by scrambling their DNA so badly that the cell can function no better than a computer whose chips have been garbled. But research on p53 now shows that radiation does not work that way. The rays damage a cell only a little. Not enough to kill it--just enough to activate p53--assuming the cell has a healthy p53. If it does not, radiation therapy might do no good and can even cause further harm. Some chemotherapies, too, work by activating p53. Tamoxifen, a chemotherapy in breast cancer, activates a cell's suicide program through p53. "Tumor cells carrying a functional p53 gene," says MIT's Jacks, "are much more sensitive to radiation and various chemotherapies than comparable cells in which p53 is nonfunctional." In those cases, oncologists are experimenting with therapies that do not rely on p53 to kill cancer cells--such as taxol for breast cancer.
The possibilities for p53-based cancer therapy are limited only by the imagination. Says Hopkins's Vogelstein, "Targeting p53--through gene therapy or other novel approaches--could bring a new dimension to cancer treatment." In September researchers led by Jack Roth of the University of Texas M. D. Anderson Cancer Center announced that they had jury-rigged viruses to carry healthy p53 genes. They injected zillions of the viruses into nine patients with lung carcinomas. In three patients the tumors stopped growing; in three more the tumor actually shrank. Even better, the p53-carrying-viruses did not have to infect every cancer cell. In a mysterious "bystander effect," even cells that didn't take up the normal p53 somehow died off. "Even if you get a gene into only a fraction of the cells, you can have an effect on the much larger tumor," says Dr. James Zweibel of the National Cancer Institute.
In another approach, Frank McCormick of Onyx Pharmaceuticals turned a broken p53 gene against the cell. He slipped a virus into mouse tumor cells. Usually, p53 keeps the virus in check. But in tumor cells with broken p53 genes, the virus replicates away, eventually bursting the cell. Since the virus spreads, explains McCormick, "you can infect just one or two cells" but still destroy the whole tumor. His next experiments will determine whether the assassin virus (technically, an adenovirus) can evade the immune system. Understanding p53 has also led to ideas for new drugs. Curt Harris of the National Cancer Institute envisions drugs that keep rogue proteins from tying up p53 like a fish in a net, or compounds that mimic the function of p53, turning on genes that halt cell growth or trigger cell suicide.
Dec. 23 marks the 25th anniversary of the National Cancer Act. It was the first salvo in "the war on cancer." Some $28 billion later, the war is far from won. Although cancer mortality is finally drop- ping (it fell by almost 3 percent from 1991 to 1995), most of the credit goes to a decrease in the rate of smoking and, to some extent, earlier diagnoses. None of the fruits of molecular biology can yet reliably cure a lung tumor, prevent a breast cancer, stop a spreading melanoma. It may be naive to think that p53 can do better. After all, some 40 percent of cancers do not seem to involve p53, for unexplained reasons. But this one little molecule already, with no help from doctors, stops incipient cancers millions of times every day. Scientists do not have to top the elegant system that nature has engineered. They just have to harness it.
The ribbonlike strands of p53 float to the double helix, where they keep a damaged cell from proliferating. But if p53 is caught in a molecular net, cancer can grow.
The p53 gene keeps tumors from forming. It either stops a damaged cell from growing into a tumor--or it kills it outright.
Every human cell (except sperm, eggs and red blood) contains 23 pairs of chromosomes.
the p53 gene is located on the short arm of chromosome 17.
If the p53 gene is healthy, it instructs the cell's protein factory to make p53 protein.
The p53 protein locks onto genes, activating the cellular-defense system.
http://www.newsweek.com/cancer-killer-175428
THE CANCER KILLER
In other families, as generation gives rise to generation, father passes to child a cleft in the chin, a love of music; mother passes to child an aptitude for dance, a curve of the cheeks. In one family, the legacy was far different. What was being handed down, unknowingly, was cancer. Or, more precisely, the mutant form of a gene whose inheritance virtually guarantees that a malignant tumor will grow. Grandfather carried the mutation. He developed pancreatic cancer at the age of 48; he died two years later. But first he passed on the mutant gene to his five children. One daughter developed breast cancer at 22 and thyroid cancer at 34. A son had a brain tumor at 54. Another daughter was diagnosed with breast cancer at 32; a year later she was dead. And that was only the first generation.
For as long as biologists have studied cancer, they have nurtured one dream. Their hope has been that, for all of the many different cancers that can eat away at a body--from the glioblastomas that creep through the brain like kudzu through Georgia, to the lung and colon cancers that envelop vital organs like a death shroud--there will turn out to be a single step that determines whether cells turn cancerous. By targeting drugs at that step, physicians would be able to stop a cell from heading down the path toward cancer just as a switchman stops a train from going down the wrong track. It turns out that a gene called p53--the gene whose useless, mutant form the luckless family passed from parent to child through the generations--may be that switch. If p53 is healthy and doing its job, it keeps the cell on the track of normalcy. But if the p53 gene is absent, damaged or tied up by other molecules, the cell goes down the track toward cancer. "p53 fulfills this long-held hope," says Dr. Bert Vogelstein of the Howard Hughes Medical Institute (HHMI) and the Johns Hopkins Oncology Center, who found, in 1989, that some 80 percent of colon cancers involve p53. "Alterations of [the p53 gene] appear to be involved, directly or indirectly, in the majority" of human cancers. All told, p53 has been implicated in more kinds of cancer than most people knew existed: 52 and counting.
Not bad for a gene that first broke on the scene in a bad case of mistaken identity. In 1979, David Lane of the University of Dundee in Scotland and Arnold Levine of Princeton University independently discovered p53, the protein that the p53 gene makes. In 1982 biologists isolated the p53 gene. But it seemed to cause, rather than suppress, cancer. Few researchers were interested in yet another one of those. It was not until 1989 that Levine and Vogelstein separately discovered p53's true colors: it was a tumor killer. With this realization, some 2,000 biologists have now dropped the date they brought to the dance--their previous research subject--and latched onto the pretty new arrival. "Our interest converged on p53 like no other molecule," recalls oncologist Richard Elledge of the University of Texas Health Science Center at San Antonio. Three years ago p53 won the science world's Academy Award, earning "Molecule of the Year" honors from the journal Science. Just last month researchers led by Nikola Pavletich of New York's Memorial Sloan-Kettering Cancer Center produced a stunning photo of p53 (above) that shows how rogue molecules can prevent it from performing its good deeds. Today there are more than 5,200 published studies on p53, and biologists are convinced that p53 is bound for even greater glory. "What I like so much about p53 is that it predicts ways to treat cancer," says Princeton's Levine. "For the first time, we can honestly translate from the lab to the clinic."
p53 acts as the cell's director of damage control. A healthy cell, usually, keeps a small number of p53 proteins around, continuously degrading them and replenishing the supply. But if something--ionizing radiation, a chemical carcinogen, chemotherapy drugs--damages a cell's DNA in a way that threatens to set it on the path to cancer, the cell switches into high alert. If everything is working right, something signals the p53 to stop degrading "and tells it that it's time to be active," says molecular biologist Carol Prives of Columbia University. The p53 supply builds up. p53 starts acting like an office clerk who, discovering a typo in an original document that is about to be copied, turns off the copier until he can fix the typo. p53 turns off the cell's copying machinery until the cell can repair its damaged DNA. To do this, p53 floats toward the cell's genes (diagram, page 46). It slips into a specific stretch of DNA. There, it triggers expression of genes nearby. These genes make proteins that directly inhibit growth of the cell. The tumor-to-be is stopped dead. Sometimes, though, p53 acts more like the clerk so disgusted with the many typos that he just trashes the document: p53 activates the cell's suicide software.
Except when it doesn't. Even good genes can go bad, and the gene that makes p53 has several ways of failing. Those failures account, directly or indirectly, for an estimated 60 percent of human cancers, including those of the breast, lung, liver, skin, prostate, bladder, cervix and colon (chart, page 45). Most often, the p53 gene goes bad by undergoing a mutation, typically a spelling mistake (FAQs, page 50). One of the 2,362 chemical "letters" (designated A, T, G and C) that make up the p53 gene changes into another letter. When the p53 gene contains such a spelling mistake, the p53 protein that the gene makes is garbled, too. And proteins are not very forgiving of errors. A single wrong letter in a crucial part of the p53 gene produces a protein with a wrong molecule; the protein is now no more able to suppress tumors than a cork is to cap a volcano.
The daughter with both breast and thyroid cancer married. It would be years before Drs. Frederick Li, of the Dana Farber Cancer Institute in Boston, and Joseph Fraumeni, now at the National Institutes of Health, would discover the silent killer--a mutated form of the p53 gene--in each and every one of her cells. Including her egg cells. She bore two daughters and a son. She passed the mutant p53 gene to both daughters. Her little boy, through the dumb luck that biology sometimes tosses up, was spared. The first little girl developed a soft-tissue cancer in her abdomen at the age of 1; she didn't live to see two candles on her birthday cake. The second daughter developed a soft-tissue cancer in her thigh, at 3.
The Li-Fraumeni families (the syndrome is named after its discoverers) inherit their p53 mutations. If the sperm or egg from which a baby grew held a mutant p53, then every single cell of the 30 trillion in her body will also harbor a mutant copy. In theory, inheriting only one mutant p53 gene, from one parent, should not be a problem as long as the child inherits a healthy p53 gene from the other parent. The healthy copy should make enough p53 to keep tumors at bay. But p53 doesn't work that way. First of all, each cell with one bad p53 gene is only "one mutation away from completely lacking the function of this critical gene," explains p53 researcher Tyler Jacks of HHMI and the Massachusetts Institute of Technology. That mutation can occur when the cell--in the liver, lungs or any other organ--makes a spelling mistake as it copies its genes before dividing into two cells. Then the cell has lost its primary defense against cancer.
But even a cell whose healthy p53 gene stays that way can be in trouble. The p53 proteins made by the genes, both good and bad, get together in groups of four to form a sinuous complex that looks like ribbon on a Christmas present. If the mutant gene is churning out mutant proteins, then each four-ribbon tangle likely has a mutant among its strands. That is enough to keep the p53 ribbon from binding to DNA and halting tumor growth.
Most p53 mutations, though, are not inherited. Instead, they arise from a copying error or an attack by a carcinogen. Bulky chemicals, such as the benzopyrene in cigarette smoke, change G to T and C to A, for instance. A single mutant gene is enough to leave a cell with no healthy, tumor-quashing p53. And just one out-of-control cell can give rise to a deadly tumor.
The only son seemed to have evaded the family legacy. But his daughter developed leukemia by the age of 5; she died soon after her 6th birthday.
THE RUSH TO STUDY P53 has left pioneers in the field with divided feelings. On the one hand, says Princeton's Levine, "it is very hard to do original experiments when there are so many smart people going after the same thing. It can be dispiriting. In 1989, when Vogelstein found so many colon cancers had p53 mutations, I moved my whole lab into p53 research. But two years ago I started half of them on different projects; it's easier to be novel in new areas. And besides, p53 is in very good hands now." And in an unusual situation for a hot field with "Nobel" written all over it, those hands are cooperating. "People share [materials] even before they publish, which is very unusual," says Moshe Oren of Israel's Weizmann Institute. "But because it's so hard to keep up with the scientific literature, you have the embarrassing situation of rediscovering something that was discovered two years before."
Still, better rediscovery than none at all. Recently researchers have focused on how p53 cancers tend to be especially aggressive. They are more often fatal than cancers in which p53 is healthy. Part of the reason may be that a mutation can do worse than simply cripple p53. Levine and colleagues find that p53 can undergo "gain of function" mutations: they actually stimulate cell division rather than suppress it. The mutant seems to turn on a new set of genes, such as those that make a cell immune to cancer drugs.
A truism of oncology holds that cancer leaves no calling card. It is thought to be impossible to know for sure what started a tumor--secondhand smoke inhaled in a lobby, a virus contracted from one's first lover. p53 promises to change that. By examining the p53 genes in a tumor biopsy, researchers can, in some cases, trace the disease back to its cause. As UT's Elledge puts it, "p53 can... be used as an archeological tool to tell us about past molecular skirmishes that touched off a cancerous war." The body count:
Cancer-causing benzopyrene in cigarette smoke primarily changes just one letter, at one spot, on the p53 gene: a G becomes T. Other compounds in smoke change C to A. In October, Texas researchers detected benzopyrene clinging right to the mutational "hot spot" that is mutated in lung cancer. "This is the closest to a smoking gun linking smoking to cancer that people have gotten," says molecular biologist Stephen Friend of the Fred Hutchinson Cancer Center in Seattle. The mutation robs the lung cell of its primary tumor-suppressing machinery. But cancer is not inevitable--"only" about 14 percent of cigarette smokers develop lung cancer. The lung cell must take one more hit before it starts growing wildly.
Two leading causes are the hepatitis-B virus and aflatoxin, a mold that grows on wet corn and other crops. Both work through p53, but in different ways. Aflatoxin causes a mutation by changing one G to a T; if the liver cell starts proliferating out of control, a hepatocarcinoma develops. The virus acts differently. It makes a protein that lurks near where p53 is made. Then it imprisons p53 and keeps it from reaching the double helix in time to stop proliferation.
If a skin cell's DNA is damaged by sunlight, p53 makes it commit suicide. But if ultraviolet light also causes a spelling mistake in the p53 gene (CC to TT), the damaged cell can proliferate unchecked.
When the human papillomavirus (HPV) sneaks into a cell of the cervix, usually during intercourse, it churns out virus proteins. One protein literally digests p53, turning it into a useless mush.
Researchers were baffled to discover that, in many breast cancers, the p53 gene looked perfectly healthy. But clearly it was not doing its job of stopping, or killing, proliferating cells. It turns out that, in at least some cases, a miscreant protein traps p53, explains Princeton's Levine. P53 can't get anywhere near the DNA that it needs to reach to save the cell. (Interestingly, during lactation of breast cells p53 is sent into exile to the cytoplasm; that way, they can proliferate enough to nurse a baby.) It is not known how often the trapping of p53 allows malignant breast cells to grow, but in another kind of cancer it is fairly common. In some 30 percent of soft-tissue tumors called sarcomas, a protein called MDM-2 snares p53 like a tuna in a net. "MDM-2 forms tight complexes with p53," says Moshe Oren, "and inactivates it."
Stephen Friend suspects that the fingerprints of other chemicals, from pesticides to industrial pollutants, will eventually be found on p53, answering how often pollutants cause cancer. Still, given the choice between knowing where a cancer came from and stopping it from going where it's going, everyone would choose the latter. Over the last two years, researchers have been making headway in using p53 to diagnose cancer early enough to allow lifesaving surgery, to determine the best therapy and--since no saga of cancer research would be complete without this-- to cure it.
The first sign of bladder cancer is often blood in the urine. That alerts doctors to screen the urine for abnormal cells. But they often do not find any until the cancer is too far along to treat. Researchers led by Hopkins's David Sidransky managed to use p53 to diagnose Hubert Humphrey's bladder cancer in a urine sample that had been stored since 1967 (Humphrey died in 1976). Using a genetic probe, they found, in a 1994 experiment, that cells in the urine contained a p53 mutation. If the diagnosis had been made in 1967, says Sidransky, it "could have changed the course of political history." Sidransky estimates that a quick and accurate urine test for bladder cancer, based on p53, is only two or three years away. Detected that early and treated--sometimes simply by nipping out the malignant cells--the cure rate is at least 90 percent.
The state of the p53 gene in a tumor can dictate the best course of treatment. For years oncologists assumed that radiation kills cells directly, by scrambling their DNA so badly that the cell can function no better than a computer whose chips have been garbled. But research on p53 now shows that radiation does not work that way. The rays damage a cell only a little. Not enough to kill it--just enough to activate p53--assuming the cell has a healthy p53. If it does not, radiation therapy might do no good and can even cause further harm. Some chemotherapies, too, work by activating p53. Tamoxifen, a chemotherapy in breast cancer, activates a cell's suicide program through p53. "Tumor cells carrying a functional p53 gene," says MIT's Jacks, "are much more sensitive to radiation and various chemotherapies than comparable cells in which p53 is nonfunctional." In those cases, oncologists are experimenting with therapies that do not rely on p53 to kill cancer cells--such as taxol for breast cancer.
The possibilities for p53-based cancer therapy are limited only by the imagination. Says Hopkins's Vogelstein, "Targeting p53--through gene therapy or other novel approaches--could bring a new dimension to cancer treatment." In September researchers led by Jack Roth of the University of Texas M. D. Anderson Cancer Center announced that they had jury-rigged viruses to carry healthy p53 genes. They injected zillions of the viruses into nine patients with lung carcinomas. In three patients the tumors stopped growing; in three more the tumor actually shrank. Even better, the p53-carrying-viruses did not have to infect every cancer cell. In a mysterious "bystander effect," even cells that didn't take up the normal p53 somehow died off. "Even if you get a gene into only a fraction of the cells, you can have an effect on the much larger tumor," says Dr. James Zweibel of the National Cancer Institute.
In another approach, Frank McCormick of Onyx Pharmaceuticals turned a broken p53 gene against the cell. He slipped a virus into mouse tumor cells. Usually, p53 keeps the virus in check. But in tumor cells with broken p53 genes, the virus replicates away, eventually bursting the cell. Since the virus spreads, explains McCormick, "you can infect just one or two cells" but still destroy the whole tumor. His next experiments will determine whether the assassin virus (technically, an adenovirus) can evade the immune system. Understanding p53 has also led to ideas for new drugs. Curt Harris of the National Cancer Institute envisions drugs that keep rogue proteins from tying up p53 like a fish in a net, or compounds that mimic the function of p53, turning on genes that halt cell growth or trigger cell suicide.
Dec. 23 marks the 25th anniversary of the National Cancer Act. It was the first salvo in "the war on cancer." Some $28 billion later, the war is far from won. Although cancer mortality is finally drop- ping (it fell by almost 3 percent from 1991 to 1995), most of the credit goes to a decrease in the rate of smoking and, to some extent, earlier diagnoses. None of the fruits of molecular biology can yet reliably cure a lung tumor, prevent a breast cancer, stop a spreading melanoma. It may be naive to think that p53 can do better. After all, some 40 percent of cancers do not seem to involve p53, for unexplained reasons. But this one little molecule already, with no help from doctors, stops incipient cancers millions of times every day. Scientists do not have to top the elegant system that nature has engineered. They just have to harness it.
The ribbonlike strands of p53 float to the double helix, where they keep a damaged cell from proliferating. But if p53 is caught in a molecular net, cancer can grow.
The p53 gene keeps tumors from forming. It either stops a damaged cell from growing into a tumor--or it kills it outright.
Every human cell (except sperm, eggs and red blood) contains 23 pairs of chromosomes.
the p53 gene is located on the short arm of chromosome 17.
If the p53 gene is healthy, it instructs the cell's protein factory to make p53 protein.
The p53 protein locks onto genes, activating the cellular-defense system.
http://www.newsweek.com/cancer-killer-175428


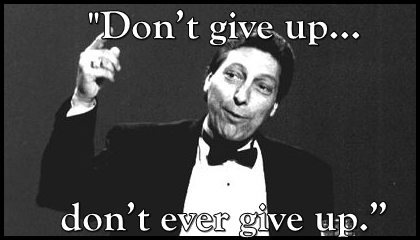